Hox protein
This is a joint project of students at La Cañada High School, La Cañada Flintridge, California USA, and students at the University of Southern California, Los Angeles, California USA, mentored by Professor Remo Rohs.
Hox Proteins Recognize the Sequence-Dependent Shape of the Minor GrooveHox Proteins Recognize the Sequence-Dependent Shape of the Minor Groove
Introduction and Biological Role of Hox ProteinsIntroduction and Biological Role of Hox Proteins
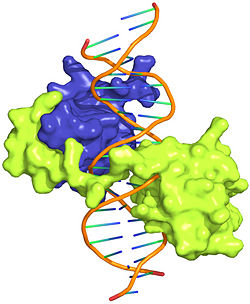
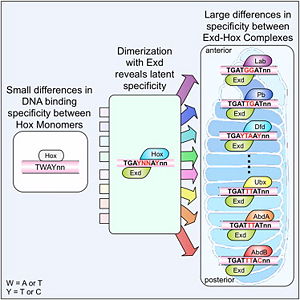
Hox proteins are transcription factors that play a key role in the embryonic development across species by activating and repressing genes. In Drosophila, eight Hox proteins are responsible for the development of different body segments of the fly, such as its antennae, wings, or legs. Hox proteins execute their distinct functions through binding to similar but different in vivo binding sites[3]. This page discusses molecular mechanisms through which Hox proteins recognize their DNA targets with very high binding specificity.
The crystal structure of a Hox-DNA complex (Figure 1) shows that the Hox protein Sex combs reduced (Scr) binds its specific in vivo site with the help of cofactors, Extradenticle (Exd)/Pbx proteins. Hox proteins can bind DNA as monomers but their binding specificity is enhanced when the co-factor is present, a principle that is called latent specificity (Figure 2). In Drosophila, for instance, eight Hox proteins bind as heterodimers with their cofactor Exd to similar but distinct target sites.
Hox proteins are expressed along the anterior-posterior axis of an embryo, thus determining the localization for the development of different body segments (Figure 2). This spatial order of expression from anterior to posterior is congruent with the location of the respective Hox genes at the chromosome, a fact known as collinearity.
Hox mutants can lead to malformations, and studying the molecular basis of how Hox proteins execute distinct in vivo functions, therefore, remains an important field of biomedical research.
Structural Description of Hox-DNA ComplexStructural Description of Hox-DNA Complex
|
Homeodomain ArchitectureHomeodomain Architecture
Both Scr (yellow) and Exd (blue) belong to the family of homeodomain proteins, which are encoded by homeoboxes. comprised of three alpha helices (Figure 3). The interface residues of both proteins are (dark purple for conserved residues vs. cyan for variable residues).
The third alpha helix of the Scr and Exd homeodomains, the so-called , inserts into the major groove where hydrogen bonds are formed between protein side chains and base pairs. An N-terminal tail forms contacts with the minor groove.
Hox Protein-Cofactor InteractionsHox Protein-Cofactor Interactions
Scr interacts with its cofactor Exd through hydrophobic interactions via a located at its N-terminal tail[4]. This interaction spans Scr's flexible N-terminal linker across the minor groove of its binding site. In the absence of the YPWM motif, Scr and Exd would not form a heterodimer.
Major Groove Base ReadoutMajor Groove Base Readout
Hox proteins achieve a large fraction of their binding specificity through . This form of protein-DNA recognition in the major groove is characterized as base readout since hydrogen bonds in the major groove can be used to distinguish between all four possible base pairs, A/T, T/A, C/G, and G/C. The Scr residues that engage in major groove base readout are . Major groove contacts are almost identical across the Hox protein family and are not sufficient to achieve specificity within this family of transcription factors.
Minor Groove Shape ReadoutMinor Groove Shape Readout
Minor groove contacts, in addition to base readout in the major groove, provide the level of specificity that contributes to distinguishing factors within the Hox family. It has been shown that minor groove contacts are essential for achieving specificity. Three side chains, of the Scr in vivo site fkh250. However, this additional level of binding specificity is not achieved through hydrogen bonds between protein side chains and functional groups of the bases. Such direct interactions are unable to distinguish A/T and T/A, or C/G and G/C base pairs due to the overlapping location of hydrogen bond donors and acceptors.
The mechanism through which these three residues recognize the DNA minor groove is called shape readout as they do not form base-specific hydrogen bonds but rather recognize the sequence-specific narrowing of the minor groove. AT-rich regions can be characterized through an intrinsically narrow minor groove, leading to enhanced negative electrostatic potential, which in turn attracts basic side chains. This shape readout mechanism was found to be broadly employed by arginine residues [5].
Biological Function of Minor Groove Binding ResiduesBiological Function of Minor Groove Binding Residues
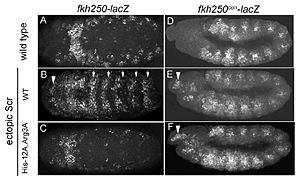
In vitro binding studies have shown that His-12 and Arg3 mutations have a large effect when exposed to the Scr specific site, whereas the effect is small when exposed to a Hox consensus site. The biological importance of both side chains becomes apparent in in-vivo experiments. Upon mutations of His-12 and Arg3 to alanine, Scr expression in a fly embryo is dramatically affected (Figure 4). In comparison to wild type Scr (A) and based on ectopic expression (B), there is only residual expression detected in
the thorax region of the double mutant when the Scr specific site is tested (C), whereas there is no apparent effect on expression in the presence of a Hox consensus site (D-F).
Recognition of Scr Specific vs. Hox Consensus SiteRecognition of Scr Specific vs. Hox Consensus Site
|
This observation can be explained based on a second crystal structure of an Scr-Exd-DNA ternary complex where the Hox-Exd hetrodimer is bound to a Hox consensus site, which is not specific to Scr. In this structure it is apparent that only and the remainder of the N-terminal linker is disordered (Figure 5).
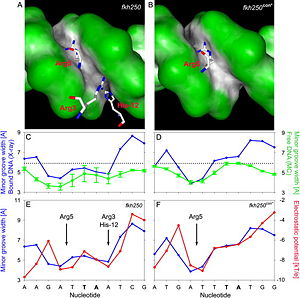
Based on the comparison of the two crystal structures of a Scr-Exd-DNA ternary complexes (Figure 6), it was found that three N-terminal residues contact the minor groove of the Scr specific site fkh250 (A) compared to only Arg5 binding the Hox consensus site fkh250con (B). In their protein-bound states, the shapes of both sites are distinct (dark gray, concave; green, convex surfaces). The distinct shapes of the two DNA binding sites, shown as minor groove width in the crystal structures of the complexes (blue plots), are already present when the protein is not bound to the DNA, with two minima in fkh250 (C) vs. one minimum in fkh250con (D), as inferred by Monte Carlo simulations (green plots). Minor groove width (blue plots) and electrostatic potential (red plots) correlate and form two binding pockets in fkh250 (E) and only a binding site for Arg5 in fkh250con (F).
High-throughput Analysis of Hox-DNA Binding SpecificityHigh-throughput Analysis of Hox-DNA Binding Specificity
Based on SELEX-seq data and a method for high-throughput prediction of DNA shape, the same pattern of two minima in minor groove width (A) was predicted for the binding sites of all anterior Hox proteins vs. a single minimum (A) for all posterior Hox proteins (dark green for narrow groove, white for wide groove). Frames highlight the regions that correspond to the minima in Figure 6. Differences in minor groove width between binding sites can be visualized in a Euclidean distance dendrogram, which forms two branches representing anterior and posterior Hox proteins (B). The differences between both groups are significant as shown by Pearson correlation (C). Remarkably, using DNA shape of their selected binding sites the eight Drosophila Hox proteins order according to their collinearity. This result, thus, indicates how Hox genes have likely differentiated throughout evolution.
Further ReadingFurther Reading
A more general discussion of structural origins of binding specificity in protein-DNA recognition has been published along with a suggestion for a new classification of protein-DNA readout modes that goes beyond the historical description of direct and indirect readout[6].
AcknowledgementsAcknowledgements
This Proteopedia page originates from the partnership of the Rohs Laboratory at the University of Southern California with La Cañada High School. This partnership was initiated by Remo Rohs and Patty Compeau in September 2011 as Bioinformatics Institute, which is part of the Institutes of the 21st Century. Advice and technical help by Proteopedia editors Eran Hodis, Eric Martz, Jaime Prilusky, and Joel Sussman is acknowledged.
ReferencesReferences
- ↑ 1.0 1.1 1.2 Joshi R, Passner JM, Rohs R, Jain R, Sosinsky A, Crickmore MA, Jacob V, Aggarwal AK, Honig B, Mann RS. Functional specificity of a Hox protein mediated by the recognition of minor groove structure. Cell. 2007;131(3):530-43. PMID:17981120
- ↑ 2.0 2.1 Slattery M, Riley T, Liu P, Abe N, Gomez-Alcala P, Dror I, Zhou T, Rohs R, Honig B, Bussemaker HJ, Mann RS. Cofactor binding evokes latent differences in DNA binding specificity between Hox proteins. Cell. 2011;147(6):1270-82. PMID:22153072
- ↑ Mann RS, Lelli KM, Joshi R. Hox specificity unique roles for cofactors and collaborators. Curr Top Dev Biol. 2009;88:63-101. PMID:19651302
- ↑ Mann RS. The specificity of homeotic gene function. Bioessays. 1995 Oct;17(10):855-63. PMID:7487967.
- ↑ Rohs R, West SM, Sosinsky A, Liu P, Mann RS, Honig B. The role of DNA shape in protein-DNA recognition. Nature. 2009;461(7268):1248-53. PMID:19865164
- ↑ Rohs R, Jin X, West SM, Joshi R, Honig B, Mann RS. Origins of specificity in protein-DNA recognition. Annu Rev Biochem. 2010;79:233-69. PMID:20334529