Student Projects for UMass Chemistry 423 Spring 2011
Instruction and signup page (1-6 below) were posted in Sandbox423 for all students to edit. Student created projects (7-18 below) on separate sandbox pages).
This sandbox is in use for UMass Chemistry 423. Others please do not edit this page. Thanks!
Spring 2011 Chem423 Team Projects: Understanding Drug Mechanisms
Project InstructionsProject Instructions
1. Team & Structure selection, due 4/1/11: All projects & names must be posted on the list below, linking to sandbox pages displaying initial structure.
- Form teams of 4 people; include both chemistry and chemical engineering majors on each team. Start by finding a protein-drug or nucleic acid-drug complex with a known structure in the pdb that interests your team. Check the list below to see if another team has already chosen this complex. If not, start a new sandbox page (just try sandbox## in the search box to find an unused number) and add a link for your team/protein to our class list below (use editing button above (Ab) or follow my model).
- Copy the message at the top of this page into your sandbox page to "reserve" your sandbox for this course.
- Find the pdb id for your protein-drug complex in the Protein Data Bank. In your sandbox page click"edit this page" (top) and follow the directions to insert your rotating structure on your page.
2. Project completion, due 4/22/11. Your proteopedia page should be organized into the following 5 required sections, with each team member responsible for one of these sections of the team project.
a. Introduction
- Introduce the protein function and the disease treated by the drug. This must be written in your own words with citations to your sources.You cannot include a copyrighted figure unless you request permission to use it.
b. Overall structure
- Describe the overall structure of your protein in words and make "green scenes" to illustrate your points. What elements of secondary structure are present (ie 5 alpha helices and 2 beta strands) and how are they organized? Below I illustrate the start of an "overall structure" section on GFP. Additional description and green scenes could illustrate the polar/nonpolar distrubution of amino acids (is the inside of the barrel polar or nonpolar?), packing of amphipathic elements, etc.
c. Drug binding site
- Describe features of the drug binding site in words and make "green scenes" to illustrate your points. Show the interactions that stabilize binding of this molecule to the protein (ie H bonds).
d. Additional features
- Describe and use green scenes to illustrate additional features of the protein. What you do here depends on what information is available. If a structure of the protein-substrate complex is available, you could compare protein interactions with the substrate vs. with the drug. If the drug is a transition state inhibitor, explain and illustrate that (eg include a reaction scheme with structures of the substrate, transition state and product).
e. Credits -- at the end list who did which portion of the project:
- Introduction -- name of team member
- Overall structure -- name of team member
- Drug binding site -- name of team member
- Additional features -- name of team member
f. References This will include the published paper that describes your structure (the reference associated with your pdb code). You will get much of your information about specific interactions to look for and highlight in the structure from this reference (which is much easier than trying to find these on your own with no guidance!).
3. In-class presentations, to be announced
ExampleExample
This is a complex between a macromolecule and its ligand (but this ligand is not a drug) that illlustrates the use of green scenes:
|
Asp Receptor Ligand-binding domain
Overall structure
The ligand binding domain of the aspartate receptor () ) is a dimer of two 4-helix bundles that is shown here with the bound.[1] In this the N and C termini are at the bottom of the structure; this is where the connections to the transmembrane helices have been truncated.
Ligand binding site
Interactions that stabilize ligand binding[2] include hydrogen bonding from Tyr149 and Gln152 backbone carbonyls and Thr154 sidechain OH to the and hydrogen bonding from the sidechain nitrogens of Arg64, Arg69, and Arg73 to the two .
References
- ↑ Yeh JI, Biemann HP, Pandit J, Koshland DE, Kim SH. The three-dimensional structure of the ligand-binding domain of a wild-type bacterial chemotaxis receptor. Structural comparison to the cross-linked mutant forms and conformational changes upon ligand binding. J Biol Chem. 1993 May 5;268(13):9787-92. PMID:8486661
- ↑ Milburn MV, Prive GG, Milligan DL, Scott WG, Yeh J, Jancarik J, Koshland DE Jr, Kim SH. Three-dimensional structures of the ligand-binding domain of the bacterial aspartate receptor with and without a ligand. Science. 1991 Nov 29;254(5036):1342-7. PMID:1660187
Project Teams, Topics, Links, and Presentation DatesProject Teams, Topics, Links, and Presentation Dates
C See comment on your page.
Example (but not a drug complex): Lynmarie Thompson, ..., ..., ... - Asp receptor in complex with Asp (above)
Monday 4/25/11
Nick DeGraan-Weber, Jackie Dorhout, Rachael Jayne, Mike Reardon - flu neuraminidase in complex with tamiflu
John Hickey, Josh Drolet, Josephine Harrington, Andrea Simoni - influenza M2 proton channel
Wednesday 4/27/11
Brittany Forkus, Katie Geldart, Elizabeth Schutsky, Breanna Zerfas - Beta Adrenergic GPCR
Lucia Tringali, Shaina Boyle, Jaclyn Somadelis , Dany Mbakop -- HIV Protease
Andy Kim, Zach Brentzel, Tyler Vlass, Zach Hitzig -- Acetylcholinesterase
Friday 4/29/11
Varun Chalupadi, Anthony Laviola, Tiffany Brucker, Alan Stebbins - cyclooxygenase sandbox25
Inna Brockman, Robert Nathan, Sarena Horava, Nick Cadirov - p38 kinase sandbox713
David Peltier, Donald Einck, Ethan Leighton, Chris Coakley - Rituximab Fab sandbox99
Monday 5/2/11
Max Moulton, Sally Stras, Jordan Schleeweis, Anh Huynh -- HIV reverse transcription Sandbox77
Chris Brueckner, Daniel Roy, John Clarkson, Justin Srodulski -- Ketamine in binding complex with NMDA receptor sandbox42
Lyes Khendek, Paul Breslin, William Rowley, Joe Perito, Ashley Rivera - G-Quadruplex sandbox888
Students looking for group membersStudents looking for group members
Each group should contain at least one person from a different primary major (typically Chemistry or Chemical Engineering) than the rest.
List yourself + your major, list partial groups looking for members, list your complex if you have chosen one. Contact others to form a group.
4/1/11 update by Prof Thompson: The remaining students can go ahead and form teams regardless of major.
Luis Cristian, Chem major, lcristia@student.umass.edu - looking to be in a group with chem eng
Help EditingHelp Editing
Hint: Ctl-click or right-click on links below and select "Open Link in New Window"
Start with Help in the navigation box on the left. Some things I've found useful:
- Follow the step-by-step written Primer.
- For step-by-step instructions on creating example scenes, try Proteopedia:DIY:Scenes.
- For editing help, try Help:Editing. Guidelines for avoiding plagiarism are listed here: Proteopedia:Guidelines for Ethical Writing.
- You can use the edit button on any page to find out how other users created effects that you see in the text (not the scenes).
[General help with Wiki editing], plus more [Wiki Text examples]
Some of the above are for help editing Wikipedia pages, but the syntax is mostly the same. Proteopedia ADDS protein stuff to the WikiMedia markup language, which powers both WEB sites.
Questions & AnswersQuestions & Answers
Here is a place to post questions and answers for each other about how to do things in Proteopedia:
A very useful color scheme is "chain" which colors separate proteins or DNA strands in different colors (first select all protein or DNA).
Anyone know what format we should be putting our references in?
For references, follow the format used in the example on the Asp receptor and they will be put in automatically. You just find out the PMID code (listed in pubmed for example) and insert it into the following, at the place where you want the reference cited (click edit to see what is actually inserted here). [1] You also need to add the section:
References
- ↑ Yeh JI, Biemann HP, Pandit J, Koshland DE, Kim SH. The three-dimensional structure of the ligand-binding domain of a wild-type bacterial chemotaxis receptor. Structural comparison to the cross-linked mutant forms and conformational changes upon ligand binding. J Biol Chem. 1993 May 5;268(13):9787-92. PMID:8486661
Hey guys this is just a useful tip:
If you get an xml error after you try to save your changes it is due to the green scene coding. Our group experienced this issue and it would not let us access our sandbox. In order to fix this go back (or find the page to edit in your history) and delete the green scene code that was just entered. Then save the page and you should be back to your sandbox. This may be trivial to many, but just throwing it out there.
To highlight some interesting portion of your protein: Under the selections tab, you can "limit to residue numbers." So for example enter in 60-65, then click "replace selection" below. Then if you go to the colors tab you can pick a color for just the residues you have selected. If it is a loop or if they are hard to see you can go to the representation tab and set selection to ball and stick or spacefill.
It is also useful to click the "selection halos:" box under the picture. That shows you what you have in your selection.
Flu NeuraminidaseFlu Neuraminidase
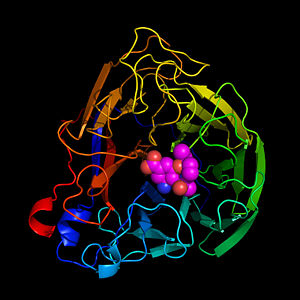
IntroductionIntroduction
Neuraminidase protein is one of two glycoproteins that coats the envelope of the influenza virus. Neuraminidase's particular function is the removal of sialic acid from the host cell, allowing the replicated influenza viruses to escape the host cell and spread to other cells.
Tamiflu, a drug designed to combat influenza, binds to and inhibits the function of neuraminidase, disabling its function and consequently disallowing the influenza virus to spread between cells.
Influenza is a disease caused by the various species and genera of the influenza RNA virus. The disease, commonly referred to as "the Flu," is highly contagious and travels around the world in seasonal epidemics. It is responsible for the deaths of between 250,000 and 500,000 people each year, and these numbers can sometimes reach the millions in particularly pandemic years. The virus is particularly lethal to people with weakened immune systems, since the virus can enable the spread of dangerous secondary infections such as pneumonias within the host.
The typical symptoms of the Flu include chills, fever, a sore throat, muscle pains, severe headache, coughing, and weakness or fatigue, as well as general discomfort.
Overall StructureOverall Structure
Influenza (flu) Neuraminidase is a homotetramer, with four identical subunits. Each subunit consists mostly of antiparallel beta sheets and three alpha helices, with a beta-propeller folding pattern (). As shown, the alpha helices lie toward the center of the protein.
The is a pocket on the surface of the protein, lined with highly conserved residues. Shown is the ligand Oseltamivir (Tamiflu) bound to one of the four subunits. When Tamiflu is bound, it tightly blocks the virus release sites on nonresistant strains of flu neuraminidase because it binds with the hydrophilic residues exposed on the surface.
Different conformations account for drug resistance of flu neuraminidase. 2hu4 is the “closed” conformation of the wild type 3hu0. For example, a mutation at the site H274Y, on the closed-form wild type, causes a change in the usual binding site for Tamiflu, possibly affecting resistance.
Research on the structures of the different mutants of flu Neuraminidase suggests that the slight change in the binding site affects the drug resistance of the molecule. In a specific case presented by Wang , et al., the H5N1 mutant being observed has a hydrophobic Tyr347 compared to the hydrophilic Asn347 of the wild type and closed form. Since Tamiflu binds to hydrophilic regions of the pore, this change has the potential to greatly affect the binding affinity, hence the mutant’s resistance to oseltamivir.
Drug Binding SiteDrug Binding Site
Flu neuraminidase is a homotetramer, and each of the four protein chains has a catalytic site. The catalytic sites are shown with Tamiflu shown in purple.
Tamiflu was designed specifically to fit to the binding site of neuraminidase by induced fit. The binding site contains a loop of residues 147-152. When it binds at the active site, Tamiflu pulls a loop made of Asp151 and Glu119 closer to the inhibitor, thereby enclosing the Tamiflu inhibitor.
There are five specific residues that are in contact with the Tamiflu substrate - Arg292, Glu276, Arg152, Arg371, and Arg118. They are in the active site.
Tamiflu does not work on all types of the flu virus, and some are becoming immune to it. However, it was recently discovered that one of the subunits has a larger surface-accessible cavity in the substrate binding region. The larger end of this cavity is not in contact with Tamiflu when the substrate is bound. This presents an opportunity to design another drug with greater specificity to this pocket.
Additional FeaturesAdditional Features
Template:STRUCTURE 2hu4 Neuraminidase breaks the groups from glycoproteins. from on the virus attaches to the sialic acid groups. The virus is from the cell and is released when neuraminidase breaks the sialic acid groups. This will allow flu replication to happen and a neuraminidase inhibitor, such as Tamiflu, is required to stop neuraminidase. The interaction of Tamiflu with N1 neuraminidase is shown with this (1). Tamiflu in this model is bound when it is visible and the loop in moved in towards the drug through induced fit.
When neuraminidase breaks the sialic acid groups, the virus (virion) is released. This will allow replication of the virus. The inhibitor prevents the breaking of the sialic acid groups, so no virion is released and replicated.
CreditsCredits
Introduction - Mike Reardon
Overall Structure - Rachael Jayne
Drug Binding Site - Jackie Dorhout
Additional Features - Nick DeGraan-Weber
ReferencesReferences
(1) Martz, E.; Hodis, E.; Canner, D.; Samish, I.; Prilusky, J.; Goodsell, D. S.; Strong, M. Avian Influenza Neuraminidase, Tamiflu and Relenza. http://www.proteopedia.org/wiki/index.php/Avian_Influenza_Neuraminidase,_Tamiflu_and_Relenza 2011.
(2) Influenza (Seasonal). World Health Organization. http://www.who.int/mediacentre/factsheets/fs211/en/ 2011
(3) "Influenza: Viral Infections: Merck Manual Home Edition". http://www.merck.com/mmhe/sec17/ch198/ch198d.html 2011
(2) OCA 7nn9 - Native Influenza Virus Neuraminidase Subtype N9. http://www.proteopedia.org/wiki/index.php/7nn9 2011.
(3) OCA 2hu4 - N1 Neuraminidase in Complex with Oseltamivir 2. http://www.proteopedia.org/wiki/index.php/2hu4 2011.
(4) Goodsell, David. Molecule of the Month: Influenza Neuraminidase- RCSB PDB. http://www.rcsb.org/pdb/static.do?p=education_discussion/molecule_of_the_month/pdb113_1.html 2009.
(5) Russell, R.J.; Haire, L.F.; Stevens, D.J.; Collins, P.J.; Lin, Y.P.; Blackburn, G.M.; Hay, A.J.; Gamblin, S.J.; Skehel, J.J. N1 neuraminidase in complex with oseltamivir 2. http://www.rcsb.org/pdb/explore.do?structureId=2hu4.
(6) Garcia-Sosa, Alfonso; Sild, Sulev; Maran, Uko. Design of Multi-Binding-Site Inhibitors, Ligand Efficiency, and Consensus Screening of Avian Influenza H5N1 Wild-Type Neuraminidase and of the Oseltamivir-Resistant H274Y Variant. Journal of Chemical Information and Modeling. http://pubs.acs.org/doi/full/10.1021/ci800242z. 2009.
(7) Wang, Shu-Qing; Dub, Qi-Shi; Huang, Ri-Bo; Zhang, Da-Wei; Chou, Kuo-Chen. Insights from investigating the interaction of oseltamivir (Tamiflu) with neuraminidase of the 2009 H1N1 swine flu virus. http://www.scirp.org/kcchou/papers/BBRC_2009_H1N1_Tamiflu.pdf. 2009.
(8) Neuraminidase Inhibitors. http://www.fluwiki.info/pmwiki.php?n=Consequences.NeuraminidaseInhibitors. 2009.
(9) Investor Update. http://www.roche.com/investors/ir_update/inv-update-2009-09-13.htm. 2009.
Influenza M2 Proton ChannelInfluenza M2 Proton Channel
|
IntroductionIntroduction
Influenza A, better known as the flu, an infection of the nose, throat, and lungs caused by the influenza virus. Basic symptoms include aches, chills, fever, loss of energy and dizziness, but complications can include pneumonia, encephalitis, bronchitis, and death—about 36,000 people every year die of complications from the flu (CDC).
The M2 protein is a proton-selective ion channel protein that plays an important role in the life cycle of the influenza A virus. The channel itself is a homotetramer with four identical M2 units, where each M2 protein is a helix stabilized by two disulfide bonds. At a low pH, the channel allows hydrogen ions to enter the viral particle form the endosome, effectively lowering the pH on the interior of the virus. This in turn causes the dissociation of the viral matrix protein M1 from the ribonucleoprotein, a critical step in “uncoating” the virus to introduce its contents to the cytoplasm of the host cell (Stouffer). The M2 protein itself consists of three major protein domains: a stretch of 24 amino acids on the N-terminal end that are exposed to the external environment, 22 (largely hydrophobic) amino acids in the transmembrane region, and 52 amino acids on the C-terminal end which are exposed to the inside of the viral particle (Schnell).
The function of the M2 channel can be inhibited by the antiviral drug Amantadine, an inhibition that effectively blocks the virus from taking over the host cell. Amantadine inhibits the replication of influenza A viruses by interfering with the uncoating of the virus within the cell. Amantadine is an M2 inhibitor that blocks the ion channel formed by the M2 protein that spans the viral membrane. By blocking this channel, Amantadine effectively prevents the acidification and subsequent release of viral elements into the host cell. Unfortunately, the M2 gene is susceptible to mutations. When one of five (of the 22) amino acids in the transmembrane region is suitably substituted, Amantadine no longer binds in such a way that would block the motion of the protons into the virus. As of 2009, the CDC noted that a full 100% of Influenza A viruses of types H3N2 and 2009 pandemic flu samples cultured in the United States showed a resistance to Amantadine (CDC).
Overall StructureOverall Structure
The M2 proton channel , made up of 97 residues, is a homotetramer transmembrane protein made up of 4 helices. The amino terminus of the protein is exposed to the outside environment, while the carboxy terminus is exposed to the internal environment. At pH 7.5, form the N-terminus. create a transmembrane helix which forms a channel. Furthermore, residues 47-50 create a ‘short flexible loop,’ and residues 51-59 form a C-terminal amphipathic helix (Schnell).
The loop created by residues 47-50 at the C-terminus connects the amphipathic helices to the transmembrane domain. The amphipathic helices lie perpendicular to the transmembrane helices. These amphipathic helices form a base that is resistant to changes in pH and therefore acts to stabilize the protein. It should be noted that the transmembrane helices are left handed while the amphipathic helices forming the base are right handed. These amphipathic helicves are also arranged head to tail (Schnell).
The pore created by the four tansmembrane helices is constricted at the N-terminus by the methyl groups on Val 27. On the other end of the pore, interactions between Trp41 also create a blockage. The four helices are packed so tightly that van der Waals forces are created between the indole rings of Trp41. This forms a gate. Together, the interactions between Trp41 and Val27 block the passage of water through the pore. Furthermore, hydrogen bonds between Asp44 and Trp41 stabilize the gate. When the pH is lowered, the imidazole rings of His37 are protonated and the helices undergo electrostatic repulsion. This in turn breaks the Asp44 and Trp41 interactions and the gate will open. As previously mentioned, the base created by the amphipathic helices prevents the protein from dissociating. However, cysteins at the N-terminus create disulphide bonds that also act to prevent dissociation (Schnell).
Drug Binding SiteDrug Binding Site
Amantadine binds with high affinity to a site in the M2 protein spanning five residues: Leu 26, Val 27, Ala 30, Ser 31, and Gly 34 (Cady). This high affinity is seen at pH’s closer to neutral. In lower pH’s the protein is only somewhat bound to amantadine. Therefore, when determining the mechanism by which amantadine blocks the channel experiments must be conducted at neutral pH. to the M2 protein is illustrated for viewing of the bonds.
When amantadine isn't present, the created in the M2 complex is open, allowing viral particles to pass through. In the presence of amantadine, this pore is , which prevents entry of the viral particles.
Additional FeaturesAdditional Features
– His37 has been found to exhibit significant proton selectivity. This suggests that His37 is involved in the opening of the conductive channel. The channel is non-conductive when His37 is not protonated, and conductive when it is in the protonated state (Pielak/Chou).
– Replacing Trp41 with a substitute amino acid results in increased channel current in in both directions. As such, the Trp 41 site is important to directional selectivity, in that it regulates the direction of the proton channel flow. This is accomplished in part because it forms a ring that prevents water from entering the channel and reaching the His37, and thus deprotonating if the pH is high, from the C-terminus(Pielak/Chou).
In addition, the proton transport channel is used to equalize the pH in the channel with that of the cytoplasm in the host cell. This prevents rearrangement of the haemmagglutanin during its transport to the host cell. (Schnell)
ReferencesReferences
Cady, S.D., Schmidt-Rohr, K., Wang, J., Soto, C., DeGrado, W.F., Hong, M. "Structure of the amantadine binding site of influenza M2 proton channels in lipid bilayers," (2010) Nature 463: 689-692.
Stouffer AL, Acharya R, Salom D, Levine AS, Di Costanzo L, Soto CS, Tereshko V, Nanda V, Stayrook S, DeGrado WF (2008). "Structural basis for the function and inhibition of an influenza virus proton channel". Nature 451 (7178): 596-9
Schnell JR, Chou JJ (2008). "Structure and mechanism of the M2 proton channel of influenza A virus". Nature 451 (7178): 591–5.
Pielak RM, Schnell JR, Chou JJ: Mechanism of drug inhibition and drug resistance of influenza A M2 channel. Proc Natl Acad Sci, 106(18):7379-84 (2009).
"CDC Recommends against the Use of Amantadine and Rimantadine for the Treatment or Prophylaxis of Influenza in the United States during the 2005–06 Influenza Season". CDC Health Alert. Centers for Disease Control and Prevention
Hay AJ, Wolstenholme AJ, Skehel JJ, Smith MH. The molecular basis of the specific anti-influenza action of amantadine. EMBO J 1985; 4: 3021-4.
Stephenson I, Nicholson KG. Influenza: vaccination and treatment. Eur Respir J 2001; 17: 1282-93.
Pielak RM, Chou JJ. "Infuenza M2 proton channels". BBAMEM-80262; No. of pages: 8; 4C: 2, 3, 5, 6.
CreditsCredits
Introduction -- Josephine Harrington
Overall structure -- Andrea Simoni
Drug binding site -- Joshua Drolet
Additional features -- John Hickey
Beta-1 Adrenergic GPCRBeta-1 Adrenergic GPCR
| |||||||
2y01, resolution 2.60Å () | |||||||
---|---|---|---|---|---|---|---|
Ligands: | , , | ||||||
Related: | 1dep, 2vt4, 2y04, 2y00, 2y03, 2y02 | ||||||
| |||||||
Resources: | FirstGlance, OCA, PDBsum, RCSB | ||||||
Coordinates: | save as pdb, mmCIF, xml |
IntroductionIntroduction
G-Protein Coupled Receptors (GPCRs) are a type of transmembrane protein that are used to pass extracellular signals into the interior, or cytoplasm, of the cell. The signal is passed to the interior of the cell by a conformational change that occurs in the G-protein on the cytoplasmic side of the cell, due to the binding of a ligand to a receptor on the extracellular side of the cell. The protein is called a G-protein because its activity depends on the binding of a guanyl nucleotide.
The signaling begins with a ligand and causes the conformational changes necessary for the G-protein to activate another transmembrane protein called andenylate cyclase. This transmembrane protein is activated by the binding of the guanosine triphosphate (GTP)-bound alpha subunit of the G-protein. The cytoplasmic G-protein is heterotrimeric, meaning that it has : alpha (purple), beta (blue) and gamma (yellow). Upon binding of the extracellular ligand the heterotrimer breaks into the beta-gamma complex and the activated alpha subunit, both of which are membrane anchored. When the GTP binds the alpha subunit the affinity of the beta-gamma complex for the alpha subunit decreases, so these two domains of the G-protein separate. The binding of the activated alpha subunit to the adenylate cyclase protein triggers the formation of cAMP from adenosine triphosphate (ATP), in which cAMP is a mobile signal transducer that can act as an activator of other proteins, often kinases, within the cell upon binding events. The small molecule cAMP is formed for as long as the activated alpha subunit of the G-protein in bound to adenylate. Also, since one ligand binding event can activate multiple G-proteins, and one adenylate cyclase protein can activate the formation of cAMP molecules, the effect of the signal is amplified within the cell.
The alpha subunit is deactivated by a spontaneous hydrolysis reaction that converts GTP into GDP. This allosteric deactivation allows the heterotrimer reform at the base of the GPCR membrane protein. The deactivation process includes the unbinding of the extracellular ligand to return the receptor to its deactivated state, and the dephosphorylation of certain amino acids in the carboxy-terminus tail of the GPCR. The GPCRs can be reactivated when a ligand binds the extracellular receptor again, which is mostly a function of the concentration of ligand in the extracellular space.
There are multiple ligands and small signaling molecules that are utilized in the case of GPCRs. Some of the ligands include epinephrine, norepinephrine, adenosine, and GABA. Some of the small molecules include IP3 and DAG. Each ligand stimulates a different pathway and therefore also calls for the use of second messengers (small molecules) in intracellular signaling.[1]
GPCRs are currently the target of many drugs because of their allosteric sites that are separate from direct chemical binding sites of the protein. This allows for conformational changes of the protein without competitive binding at the chemical site. Since binding an allosteric site is reciprocal, the types of cooperativity vary based on the chemistry of the ligands. There are three main advantages to using allosteric binding sites on GPCRs as drug targets: (1) the drug is saturable and therefore can only affect the cells to an upper or lower limit, (2) the response of the cell can change with time and space when using an allosteric modulator, and (3) the potential increased selectivity of the binding site. There are multiple binding sites and ligands that can effect the activity, or non-activity, of the protein. In this case, we are describing the interaction of the drug dobutamine with the of a G-protein coupled receptor.
Overall StructureOverall Structure
Since this protein is membrane bound, determining the structure was very difficult. Transmembrane proteins are difficult to look at because they are complicated to separate and crystallize. For these reasons, it took about 20 years of studies to determine the structure of β-adrenergic GPCR; the structure was even mutated to increase its thermal stability so that it could be examined[3]. This protein is made of , each represented in a different color in the scene. Just as is true of most GPCRs, the dimers are each made up of seven , all of which must span the membrane; the α helices are connected by external and internal loops and are connected in an form[4]. For these α helices to be stable, their middle must be made up of mostly hydrophobic amino acids while their ends are hydrophilic. In this , hydrophobic amino acids are colored grey while polar amino acids are purple. Though it is shown that some polar amino acids exist on the middle of the helices, they are also mostly on the interior of the helix. This keeps them from being exposed to the lipid membrane and destabilizing the protein. It is also required that all of the hydrogen bonding sites in the α helices are satisfied, so that there are no unfavorable interactions between the lipid inside of the membrane and the protein. As can be seen, the (represented in white) are all between the amino acids in the α helices and not from interactions with the ligand. The ligand to the protein must also have these similar qualities, or else it would not be able exist within the membrane. As seen in this , the ligand consists of hydrocarbon chains and rings in the center with nitrogen (blue) and oxygen (red) atoms only existing on the ends. This gives the ligands a very similar structure to the membrane, with a hydrophobic center and polar ends. Most of the ligands exist , allowing them to participate in binding along with the protein. [5]
| |||||||
2y01, resolution 2.60Å () | |||||||
---|---|---|---|---|---|---|---|
Ligands: | , , | ||||||
Related: | 1dep, 2vt4, 2y04, 2y00, 2y03, 2y02 | ||||||
| |||||||
Resources: | FirstGlance, OCA, PDBsum, RCSB | ||||||
Coordinates: | save as pdb, mmCIF, xml |
Drug Binding InteractionsDrug Binding Interactions
A common technique employed by researchers in the field of modern drug development is to design synthetic ligands that structurally and biochemically mimic natural molecules to induce a desired physiological response. With respect to the beta-adrenergic receptors, several drugs have been created that function to activate or inhibit the receptors for clinical implications such as the treatment of asthma, hypertension, and cardiac dysfunction. In particular, activation of the beta1-adrenergic receptor results in increased cardiovascular output and several drugs including, the total agonists, carmoterol and isoprenaline, and the partial agonists, salbutamol and , have been designed to take advantage of this feature. The classification as, full agonists, partial agonists, or antagonists is dependent on whether the ligand functions to activate, reduce, or inhibit the natural cellular responses, respectively. However, it is interesting to notice that each of these three classifications has significant effects on the interactions between the ligand and the drug-binding site.

When any of the aforementioned drug agonists bind to the beta-1 receptor, they fit into the natural catecholamine binding-pocket and are capable of inducing three structural changes that result in the protein's overall transition to an active state conformation. The first, and most important, contribution to receptor activation is the of the binding-pocket by 1 angstrom that is stimulated between the alpha carbons of Asn310 and Ser211. Stabilization of the ligand in this pocket is a major factor contributing to ligand efficacy and requires strong hydrogen bonding between the secondary amine and the beta hydroxyl group of the ligand to some of the side chains of protein helices 3 and 7. However, dobutamine lacks the traditional ligand beta-hydroxyl group which, in turn, reduces its potential bonding ability from 4 H-bonds to 2. This weakens the interactions between the helices and is responsible for much of dobutamine’s only partially agonistic behavior.
The second major change that occurs upon agonist binding is the rotamer conformational shift that occurs at . This conformational shift causes Ser212 to form stabilizing hydrogen bonds with Asn310, while simultaneously inducing ligand-mediated bonding interactions between Ser211 and Asn310. These combined actions heighten the extent of interactions between protein helices 5 and 6. The final possible binding change is only observed in total agonists. Full agonists have the ability to additionally induce a conformational change at , which severs the van der waals interactions between Val 172 and Ser215 inducing a subsequent weakening of the interactions between protein helices 4 and 5. The rotameric conformational changes that result from the weakening of interaction between H4 and H5 combined with the strengthening of interactions between H5 and H6, results in a conformational change that is extremely similar to that observed in the familiar and well-studied rhodopsin protein and is believed to significantly contribute to protein activation. It is also important to notice that there are several polar and non-polar interactions that take place right outside of the catecholamine binding-pocket between ligand and protein, which are essential for the specificity of drug binding.
Additional FeaturesAdditional Features
Adrenergic receptors are the targets of catecholemines, hormones produced in the adrenal glands in response to stress. These ligands result in a wide variety of sympathetic responses depending on the type of ligand and its binding region within the protein[5]. The alpha and beta domains of the G-protein are broken down into smaller subunits that are more specifically involved in binding. Different tissues throughout the body often vary in the types of G-protein receptors found in the cells. Beta 1 adrenergic receptors that bind agonists in the beta 1 site are highly prominent in kidney and heart tissue cells. Beta 2 receptors on the other hand are found in cells in skeletal muscle, lungs, and the GI tract. Beta 3 receptors are found specifically in brown fat cells. It thus makes sense that introducing a beta 1 binding ligand such as would target heart or kidney cells [6]. In the case of dobutamine, the beta 1 site is preferentially bound and results in an increased frequency and intensity of cardiac contractions. Comparatively, beta 2 bound agonists cause smooth muscle and bronchial relaxation and are thus often used in the treatment of asthma. Agonists bound to the alpha receptors can result in vascoconstriction of veins and arteries as well as decreased activity of the smooth muscle in the GI tract [7].
The intensity of a sympathetic response can be controlled with how tightly the agonist is able to bind to its designated receptor. Agonists are broken down into three primary levels of activity; full agonists, partial agonists, and antagonists. Full agonists produce a similar response to that expected from the natural ligand (such as adrenaline or norepinephrine in this case). Dobutamine is considered a partial agonist because it results in a similar but damped response. The primary difference in the interaction of full versus partial agonists is the number of hydrogen bonds formed between the ligand and the protein[5]. Full agonists form two hydrogen bonds on the 5th alpha helix at Serine 211 and 215 whereas partial agonists only interact with Serine211. , a full agonist, has a similar structure to the native ligand adrenaline and is shown bound to the GPCR. The third category of ligands are called antagonists. Antagonists inhibit the response of the natural ligand. The term "beta blocker" refers to antagonists that specifically target the beta subunits on adrenergic receptors. Binding of a beta 1 antagonists competitively inhibits the binding of the natural ligand. This is why beta blockers are used to decrease heart rate to reduce oxygen deprivation of the heart [8]. is shown bound to the beta 1 subunit. Like the full and partial agonists, this beta blocker binds to Serine 211. It also hydrogen bonds to Aspartic Acid 121 and Asparagine 329 [9].
CreditsCredits
Introduction -- Elizabeth Schutsky
Overall structure -- Breanna Zerfas
Drug binding site -- Brittany Forkus
Additional features -- Katie Geldart
ReferencesReferences
- ↑ Berg, Jeremy M.. "Biochemistry." Ed. New York: W.H. Freeman and Company, 2007. Print. pp. 383-388.
- ↑ Christopoulos A. Allosteric binding sites on cell-surface receptors: novel targets for drug discovery. Nat Rev Drug Discov. 2002 Mar;1(3):198-210. PMID:12120504
- ↑ Warne T, Moukhametzianov R, Baker JG, Nehme R, Edwards PC, Leslie AG, Schertler GF, Tate CG. The structural basis for agonist and partial agonist action on a beta(1)-adrenergic receptor. Nature. 2011 Jan 13;469(7329):241-4. PMID:21228877 doi:10.1038/nature09746
- ↑ Rosenbaum DM, Rasmussen SG, Kobilka BK. The structure and function of G-protein-coupled receptors. Nature. 2009 May 21;459(7245):356-63. PMID:19458711 doi:10.1038/nature08144
- ↑ 5.0 5.1 5.2 5.3 DOI: 10.2210/pdb2y01/pdb
- ↑ Arcangelo V.P.; Peterson A.M. (2006). Pharmacotherapeutics for advanced practice: a practical approach. Lippincott Williams & Wilkins. pp. 205. ISBN 9780781757843. Retrieved 2011-04-21.
- ↑ http://www.adrenoceptor.com/betaintro.htm
- ↑ Khan, M. I. Gabriel (2006). Encyclopedia of Heart Diseases. Elsevier. p. 160. ISBN 0124060616. Retrieved 2010-09-10.
- ↑ http://www.pdb.org/pdb/explore/explore.do?structureId=2VT4
Acetylcholinesterase bound by TacrineAcetylcholinesterase bound by Tacrine
IntroductionIntroduction
Acetylcholinesterase breaks down acetylcholine into acetic acid and choline via a hydrolysis reaction.[1] Acetylcholine is a neurotransmitter that signals muscle contraction. If acetylcholine is not broken down, then the chemical builds up in the synapses between nerve cells and muscle cells resulting in loss of muscle function and ultimately paralysis. The enzyme’s extremely fast reaction rate (approaching the diffusion limit) for breaking one acetylcholine into its two components indicates acetylcholinesterase’s biological importance. Many natural poisons and toxins work by inhibiting this enzyme, thus, paralyzing the victim.[2]
Intentionally inhibiting acetylcholinesterase is a treatment for Alzheimer’s disease. Alzheimer’s is basically the progressive breakdown of the nervous system. Worldwide, there are an estimated 20 million individuals diagnosed with Alzheimer’s, most of whom are over the age of 65.[3] Symptoms of the disease include confusion, irritability and aggressioin, mood swings, language breakdown, long-term memory loss, and eventually loss of bodily functions.[4] To help combat nerve cell degeneration, these acetylcholinesterase inhibitors partially block the enzyme so that excess neurotransmitters remain in the synapse and strengthen the signal.[5]
The drug Tacrine, also known as Cognex, was the first acetylcholinesterase inhibitor approved to treat Alzheimer’s disease. Studies show that the drug only led to slight improvements in people who took it during early stages of the disease, but the drug did nothing to delay the onset of the disease.[6] Tacrine is not often used anymore because it has to be taken four times a day and has adverse side effects, including nausea, diarrhea, heartburn, muscle aches and headaches.[7]
Overall structureOverall structure
Acetylcholinesterase (AChE) is an monomeric enzyme. Most often, AChE forms a tetramer and binds with a molecule, collagen Q, to connect to the membrane of the neuromuscular junction. [8]. From the , it can be seen that there are 17 and 14 . There are 2 beta sheets formed from 3 anti-parallel and 11 anti-parallel beta sheets, respectively. As the shows, turns, alpha helices, and beta sheets all occupy a portion of the exterior of the protein. The means that the turns must be composed primarily of polar side chains. On the other hand, the alpha helices will be amphipathic with side chain order designated by the helical wheel; the exterior will be filled with polar side chains that can hydrogen bond with water while the inside of the alpha helix will have nonpolar, hydrophobic groups. The beta sheets must also be amphipathic, but the pattern of side chains is alternating polar and nonpolar. In addition, in order to maintain its tertiary structure, the protein has three sulfide bonds, which are covalent bonds that form between cysteine residues. The between cysteine 67 and cysteine 94 is 5.03 angstroms.
BindingBinding
The active site of Torpedo californica acetylcholinesterase (TcAChE) is buried at the bottom of a narrow, deep gorge in the enzyme, and contains a consisting of Ser200, Glu327, and His440. When complexed with tacrine (THA), the aromatic rings of sandwich the THA’s acridine ring . The phenyl ring of Phe330 lies parallel to and in contact with THA. THA is stacked against Trp-84. Its ring nitrogen is H-bonded to the main chain carbonyl oxygen of Hist-440 and it’s amino nitrogen is H-bonded to a water molecule.
Additional FeaturesAdditional Features
Huperzine A reversible binds with AChE at Ser200 but forms hydrogen bonds with Tyr130, Gly117, and Glu199.[9]
Decamethonium is similar to acetylcholine in that it contains trimethylammonium cations allowing it to bind to the nicotinic acetylcholine receptor.[10]
Soman binds to Ser200, in the active gorge. After binding the acetylcholinesterase(AChE) catalyzes the cleavage of the ether bond on the carbon side causing irreversible inhibition.[11]
Rivastigmine reversible inhibits AChE, binding at the active gorge. The AChE cleaves the rivastigmine into carbamyl moiety and NAP.[12]
ReferencesReferences
- ↑ http://www.proteopedia.org/wiki/index.php/Acetylcholinesterase
- ↑ http://www.rcsb.org/pdb/101/motm.do?momID=54
- ↑ http://www.searo.who.int/en/Section1174/Section1199/Section1567/Section1823_8066.htm
- ↑ http://en.wikipedia.org/wiki/Alzheimer's_disease
- ↑ http://www.rcsb.org/pdb/101/motm.do?momID=54
- ↑ http://en.wikipedia.org/wiki/Tacrine
- ↑ http://www.ncbi.nlm.nih.gov/pubmedhealth/PMH0000930/
- ↑ http://www.ncbi.nlm.nih.gov/pubmed/11804574
- ↑ http://www.proteopedia.org/wiki/index.php/AChE_inhibitors_and_substrates_%28Part_II%29
- ↑ http://en.wikipedia.org/wiki/Decamethonium
- ↑ http://www.proteopedia.org/wiki/index.php/AChE_inhibitors_and_substrates
- ↑ http://www.proteopedia.org/wiki/index.php/AChE_inhibitors_and_substrates_%28Part_III%29
CreditsCredits
Introduction - Tyler Vlass
Overall Structure - Zach Brentzel
Drug Binding Site - Andy Kim
Additional Features - Zach Hitzig